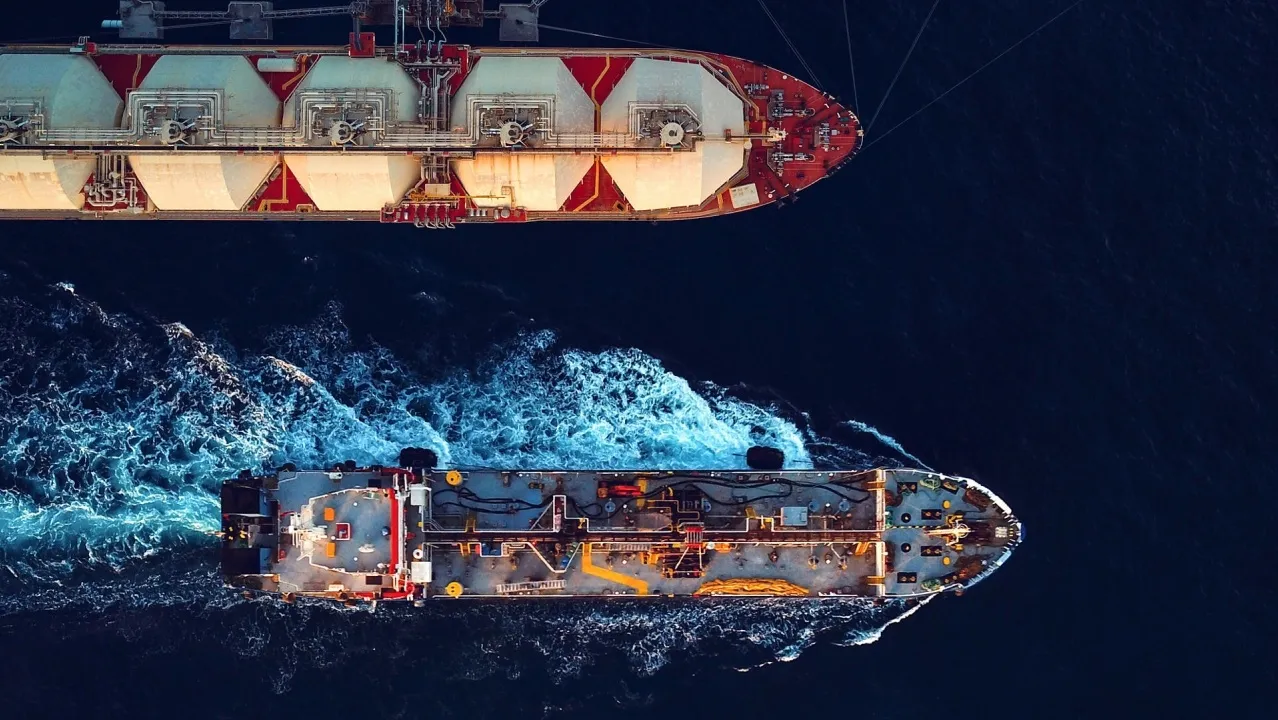
The transformative role of catalysts and catalysis
From baking bread to making paper , humans have unknowingly been harnessing the power of catalysis for thousands of years. In fact, almost everything in your daily life has been produced through the process of catalysis. Catalysts are substances that facilitate chemical reactions by lowering the activation energy required for the reaction to occur. They increase the rate of the reaction without being consumed or permanently altered in the process. Their unique properties have made them indispensable in a myriad of vital real-world applications , from fuel and pesticides to the development of life-saving pharmaceuticals.
For example, one of the most prominent catalyst-enabled reactions, the "Haber-Bosch process”, produces ammonia for fertilizer and agriculture on an industrial scale. Using catalysts tremendously lowers the cost and accelerates the production of ammonia. Even now, the Harbor-Bosch process is the major production method of ammonia.
Another example is found in catalytic converters for cars which use platinum, palladium, or rhodium to reduce emissions of toxic compounds like hydrocarbons, carbon monoxide, and nitrogen oxides by 90%.
The role of catalysis in sustainable chemistry
Though sustainability may feel like a recent buzzword, sustainable environmental practices have been firmly on the agenda since the publication of the United Nation’s (UN’s) ‘Our Common Future’ in 1987. This groundbreaking report mapped out guiding principles for sustainable development as it is generally understood today, defining the concept as “development that meets the needs of the present without compromising the ability of future generations to meet their own needs.” This definition sums up the importance of implementing sustainability into all manufactured products.
The increasing emphasis on sustainability has sparked a transformative movement towards sustainable chemistry or 'green' chemistry , revolutionizing the way we design products and processes. This innovative approach seeks to enhance the efficiency of utilizing natural resources in chemical production. Three crucial avenues are pursued to achieve this goal: minimizing energy consumption, embracing environmentally friendly chemicals, and effectively managing material life cycles. Through these methods, sustainable chemistry is paving the way for a greener and more resource-efficient future.
Catalysts play a pivotal role in our pursuit of sustainable practices, offering a valuable tool to facilitate goals. They have contributed to the creation of biodegradable plastics, reducing our reliance on harmful materials. Furthermore, catalysts are instrumental in the production of fuels and fertilizers, optimizing efficiency and minimizing waste. Harnessing the power of catalysis empowers us to achieve remarkable feats in various fields while embracing sustainability as a guiding principle.
With surging demand for catalysts, there has been an increased call for environmentally friendly products to address issues with sustainable energy production, reduce industrial emissions, and tackle climate change. Using data from the CAS Content Collection™, we will explore the current research trends in sustainable catalyst research, highlighting key advances in this field.
Making catalysts more sustainable
Noble metals such as platinum, palladium, and iridium are widely used for their desired catalytic properties, such as high stability and temperature tolerance. They are also used to facilitate a wide array of chemical reactions, including Sonogashira coupling , Suzuki-Miyaura coupling , and Heck reaction .
However, the usefulness of noble metals is hindered by their high cost and limited availability. These precious metals are primarily obtained from large amounts of low-grade ores , requiring extensive mining efforts to extract even small quantities. This extraction process not only demands significant energy input but also poses potential environmental harm. Consequently, the utilization of noble metals in catalytic applications must be carefully weighed against the environmental impact and sustainability of such practices.
The limitations posed by the economic and environmental costs of noble metals, coupled with the growing global demand for catalysts, have spurred researchers to explore alternative options, particularly non-noble transition metals like titanium, iron, cobalt, and nickel. These metals offer several advantages over their noble counterparts. Firstly, they are more abundant, ensuring a sustainable supply for catalytic applications. Additionally, non-noble transition metals are more cost-effective, making them economically viable choices. Moreover, they exhibit low toxicity levels, reducing potential hazards in both production and application. Importantly, these metals are environmentally benign, minimizing adverse ecological impacts.
While non-noble metals present a promising alternative, it is important to acknowledge that they are not without their own challenges. Non-noble metals are often more reactive than noble metals; this reactivity may lead to the degradation of catalysts (reducing their durability) and to less selective catalytic activity (which leads to byproducts, generating wastes and reducing process efficiency). Moreover, the characterization of non-noble metals can be complex and demanding (Table 1).

Nonetheless, the development of sustainable catalysts with non-noble metals is gaining traction. Insights from the CAS Content Collection reveal a large increase in publications for non-noble metal catalysts/catalysis between 2012–2022 (Figure 1).

Technologies and advancements in catalysis
Over the past several decades, an array of specialized catalysts has been developed for essential real-world applications. These catalysts broadly fall into four sub-categories: electrocatalysts, photocatalysts, homogeneous catalysts, and biocatalysts (or enzymes).

Data from the CAS Content Collection shows that electrocatalyst-related publications are dominant in sustainable chemistry using non-noble metal catalysts (Figure 2 and Figure 3). Electrocatalysts participate in electrochemical reactions either as electrodes or as catalytic materials applied onto electrode surfaces. Traditionally, platinum has been widely employed in electrocatalysis. However, its limited availability and high cost have prompted researchers to explore alternatives. One noteworthy example involves the use of nitrogen-doped graphene augmented with cobalt atoms , which has proven to be an efficient and durable catalyst for generating hydrogen from water. Approaches like this represent a significant step toward lower-cost catalysts for energy production.
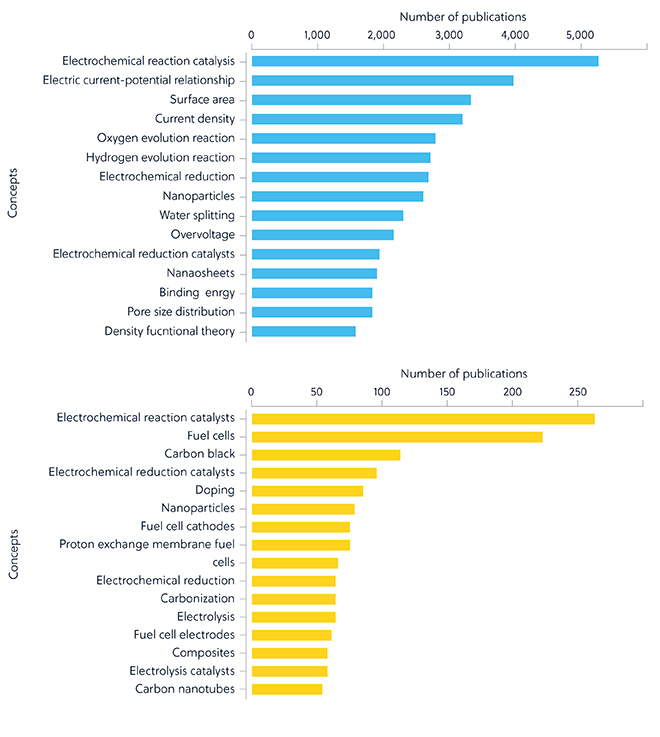
Photocatalysis is a process by which semiconductor materials absorb light energy and produce electron-hole pairs that drive reduction and oxidation reactions. It is important for solving energy and environmental problems in reactions such as water splitting to produce hydrogen and the decomposition of pollutants respectively (Figure 4). However, a major research challenge is finding non-noble metal semiconductor materials capable of splitting water using only solar energy. Several strategies are being explored in this area, including the use of co-catalysts or multi-component nanointegration .

Noble metals such as platinum and palladium are also predominant in homogeneous catalysis due to their high activity, stability, and versatility. However, finding substitutes for noble metals in homogeneous catalysts presents a complex and ongoing challenge for researchers. A key reaction facilitated by these catalysts is Sukuzi coupling. Famously, reports where authors claimed to demonstrate palladium-free Suzuki coupling were later shown to be catalyzed by low levels of palladium contaminants. However, the use of radical reaction initiators like iodine, eosin, and tetrabutylammonium iodide holds promise in this area (Figure 5).
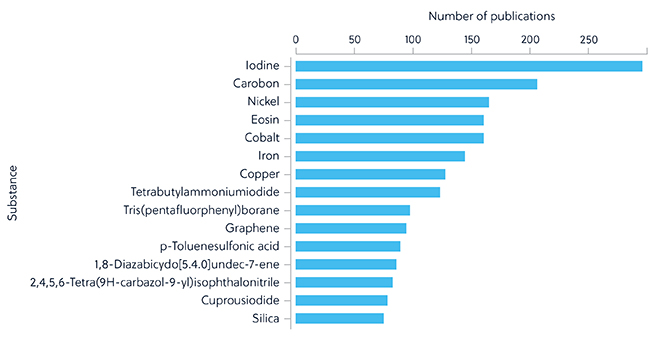
Biocatalysts, which are catalysts based on enzymes, offer a remarkable example of green and sustainable catalysts. Produced from readily available renewable feedstocks, they are organic, biodegradable, non-toxic, and can function under mild reaction conditions. A key potential application of biocatalysts is in the sustainable generation of biofuels from vegetable oils and fats by the transesterification of fatty acids with methanol. The reaction produces biodiesel (fatty acid methyl esters) and glycerol as a byproduct (Figure 6). The combination of biocatalysts and metal catalysts is also an emerging approach to achieving the sustainability of valuable molecule production.

A catalyst for change
In the wake of the UN Climate Change Conference (COP27) and the UN Biodiversity Conference (COP15), there has been a notable surge in corporate commitments to embrace more sustainable practices . As catalysts remain indispensable in the chemical industry, there is a growing impetus to explore novel catalytic concepts that can enhance the efficiency and sustainability of essential product manufacturing. Recognizing this need, the U.S. Department of Energy has made a dedicated commitment to support fundamental catalyst research.
The significant advancements in sustainable catalyst research in the past decade signify that the pursuit of environmentally friendly solutions is well underway. While the full potential of this market is yet to be realized, we anticipate a promising future for non-noble, metal-based catalysts across diverse domains encompassing organic, inorganic, and bio-based substances.
For further insights into the future of sustainable catalysis, we invite you to explore our recent publication in ChemRxV.