
While green energy’s footprint continues to increase as the fastest growing segment within the global energy mix, it still trails significantly behind conventional, high-carbon energy options due to efficiency and capacity hurdles. These limitations are preventing green energy from becoming a predominant, mainstream energy option. What other scalable, non-CO2 emitting form of energy could help us close the gap until green energy becomes a large-scale reality for us? Could nuclear energy’s carbon-free profile, proven efficiency and scalability make nuclear power a transition candidate, and possibly another viable, widely accepted energy option for the future?
In addition to their zero-emission blueprint, the approximately 450 nuclear power plants operate today at full capacity greater than 90% of the time compared to 50% for coal and 25% for solar plants. However, only 10% of the total electricity demand worldwide is supplied by nuclear power plants (Figure 1). Why hasn’t nuclear energy grown faster over the years?
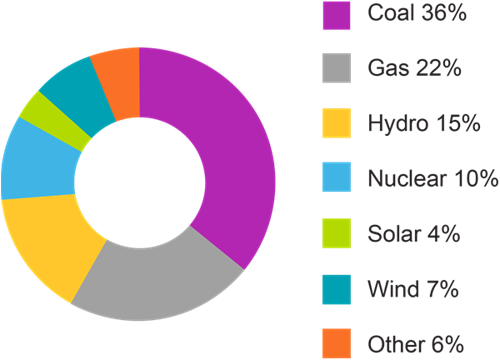
Although a proven and economical option for energy production, nuclear energy carries a controversial image due to the risks associated with radioactivity and its impact on the environment. The Chernobyl and Fukushima events reminded us that atomic fission requires flawless control and vigilance and that small incidents can turn into major catastrophes.
Nuclear Reactions and Radioactivity
With over 18,000 reactor-years of experience, nuclear reactor technology is well established, diversified, and benefits from decades of technological improvements making reactors safer, more reliable, durable, and efficient.
To generate electricity, nuclear power plants use a mixture of uranium isotopes, mainly 238U and 235U as fuel. Most commercial nuclear power plants use low enriched uranium (LEU) fuel, which is uranium with 235U enriched between 3-5%, as opposed to highly enriched uranium (HEU) which has the 235U concentrations of ~90% needed for weapon grade applications.
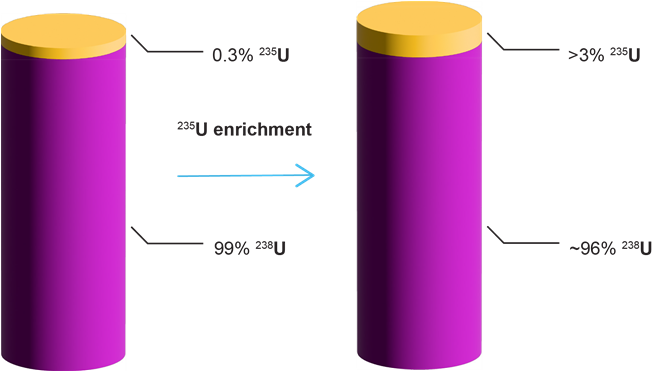
Once in the reactor as the LEU fuel, 235U and 238U take two different atomic transformation paths as Figure 3 illustrates. Through the capture of a neutron, and in the case of 238U which transmutes to fissile 239Pu, both 239Pu and 235U fission into smaller nuclei, i.e., fission products. The fission reactions each also release three neutrons and a significant amount of energy in the form of heat and ionizing radiation.
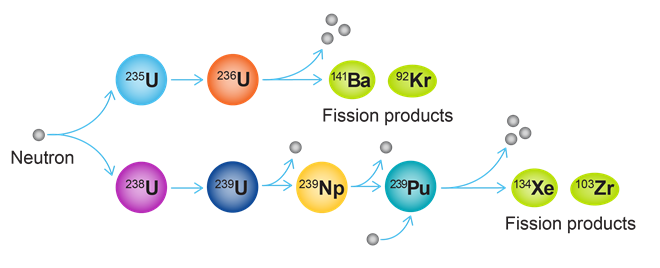
This atomic transformation or decay is a blessing and a curse. A blessing because in relation to the small quantity of fuel involved, it produces enormous amounts of energy that will be extracted through heat exchangers and high-pressure water turbines to produce electricity. It is a curse because transmutation associated atomic decay also produces ionizing radiation and particles, collectively called radioactivity. The radioactivity in the reactor for electricity production is desired, but this radioactivity persists within the fuel waste, called “spent fuel”, and can be harmful if not contained and controlled.
After 3-5 years of continuous nuclear activity in a reactor, the fuel concentration in fissile isotopes eventually falls below the minimum level to maintain a chain reaction for electricity production purposes. The spent fuel is unloaded from the reactor and categorized as “high-level” radioactive waste (HLW). HLW represents only 3% of the total radioactive waste volume but it accounts for 95% of the waste’s total radioactivity. Thus, HLW is a major focus of radioactive waste management strategies worldwide.
An average nuclear power plant with a capacity of 1000 MWe (sufficient to supply the needs of more than one million people), produces 25-30 tonnes of HLW per year and zero carbon emissions. A coal-fired plant releases 300,000 tonnes of ash and more than 6 million tonnes of CO2 to the atmosphere annually. However, reducing the footprint and the radioactive potency of nuclear waste through reprocessing and reuse of spent fuel would address a complex hazardous waste management challenge.
Nuclear spent fuel recycling options
Nuclear spent fuel reprocessing technology has existed since the late 1940’s. It is well understood and technically proven but only a few countries have invested in reprocessing. France and Russia are the two main countries reprocessing and reusing spent fuel. On average, about 95% of spent fuel waste is uranium (the majority is 238U), 1% is plutonium, and the rest are a wide variety of fission products with a lower atomic number and minor actinides (Figure 4). Spent fuel reprocessing technology allows the separation of uranium and plutonium isotopes from other actinides and fission products.
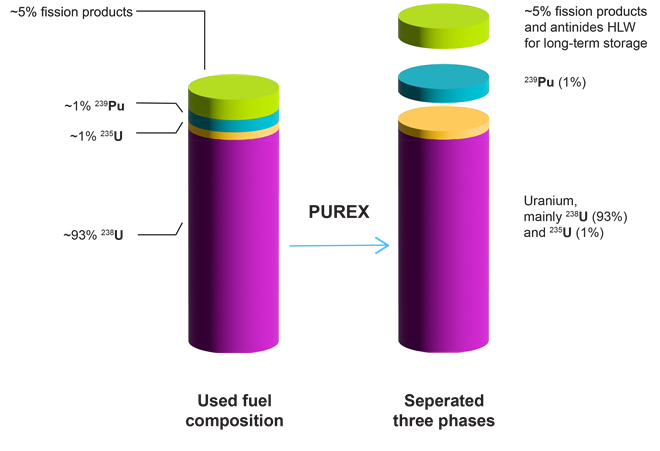
The predominant reprocessing option is called PUREX (plutonium and uranium reduction extraction). PUREX uses hydrometallurgical separation technology to separate the spent fuel into three phases:
- Uranium isotopes
- Plutonium isotopes
- Fission products with minor actinides
This third phase is considered HLW because of the presence of these minor actinides and the highly radioactive, medium-lived fission products (i.e. 90Sr and 137Cs with radioactive half-lives of about 30 years). The primary advantage of PUREX is the recycling of large amounts of usable uranium that would otherwise be considered as waste and the significant reduction in the HLW volume.
While PUREX reduces waste volume, it doesn’t address its radioactivity. Also, the separation of 239Pu from other actinides generates nuclear weapons proliferation concerns.
PUREX process variants have been proposed and implemented around the world to address HLW radioactivity and plutonium proliferation risks. These PUREX variants consist in blending 239Pu with minor actinides that would prevent it from being weaponized while creating an acceptable reprocessed actinide fuel blend. Other variants consist in blending uranium, plutonium, and all transuranics (elements with a higher atomic number than uranium) together, leaving fission products as the only waste.
HLW recycling makes sense when one considers that more than 90% of the uranium is “unburned” when spent fuel rods are unloaded from the reactor. Recycling unused uranium and plutonium allows the generation of ~25-30% more electricity. At the end of 2020, 400,000 tonnes of used fuel had been generated globally from commercial nuclear power reactors, of which about 120, 000 tonnes (30%) has been reprocessed and reused as nuclear fuel.
Advances in nuclear reactor designs
Recent advances in the design of nuclear reactors have improved energy production’s efficiency and safety. The CAS Content Collection™ shows a significant increase in patent and journal activity since 2018 indicating a renewed interest, primarily driven by organizations in Asia (Figures 5a and 5b).
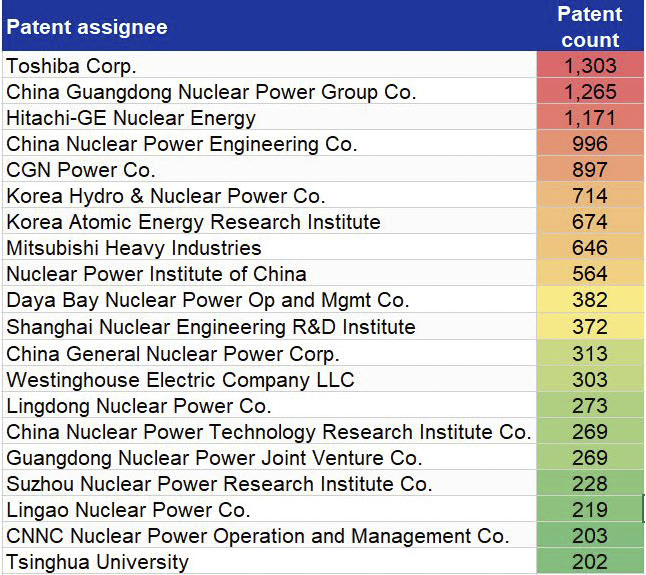
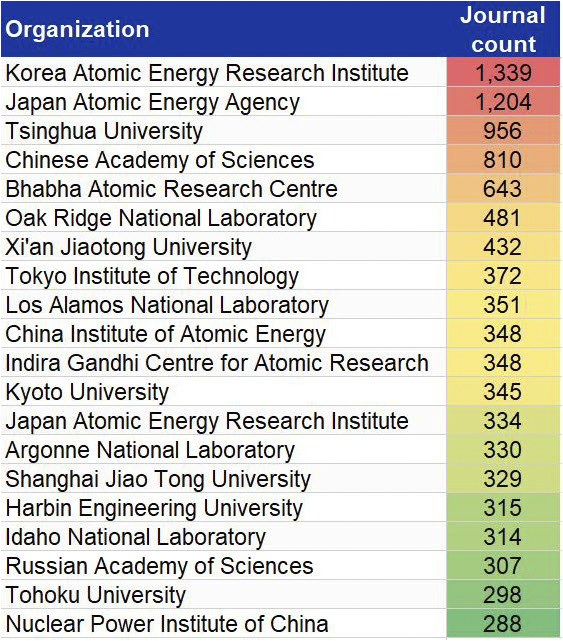
Figure 6 shows the publication volume associated with new, advanced nuclear reactor designs. The data confirms increasing research activity around these new nuclear reactor technologies.
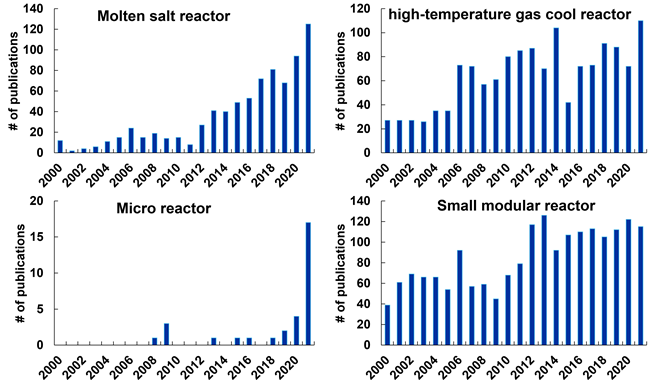
Nuclear Energy's Future Potential
The renaissance of nuclear energy has been an enduring theme, but several obstacles and challenges still make it difficult for nuclear energy to fulfill the hope and promise it generated decades ago. The large upfront capital, changing regulations, cost overruns, and political polarization have made nuclear power plant deliveries a decade-long, tortuous journey. This has been a serious deterrent for governments and investors to consider nuclear energy even if its advantages and potential are proven and undeniable. A recent Wall Street Journal article also addresses some of these challenges as well as recent developments in the field of nuclear energy technologies.
The need for carbon-free sources of energy, advances in new reactor technologies, and new spent fuel recycling and reuse alternatives could propel nuclear energy as a key tool in the arsenal to combat the global climate change challenge.
Acknowledgement to Elaine McWhirter for scientific consultation.
References for Nuclear Animation
IAE, World Energy Outlook. https://www.iea.org/reports/world-energy-outlook-2022 (accessed 2023-01-09)
World Nuclear Association. https://world-nuclear.org/nuclear-essentials/how-can-nuclear-combat-climate-change.aspx (accessed 2022-09-09)
NEK. https://www.nek.si/en/longevity-for-sustainability/production-performance/high-energy-density-of-uranium-is-one-of-key-advantages-of-nuclear-energy (accessed 2022-09-09)
World Nuclear Association. https://www.world-nuclear.org/information-library/nuclear-fuel-cycle/fuel-recycling/processing-of-used-nuclear-fuel.aspx (accessed 2022-09-09) IAE,
World Energy Outlook. https://www.iea.org/reports/world-energy-outlook-2022 (accessed 2023-01-09)